latest from the magazine
latest journal issue
I
n this essay, we explore the nexus of relations between national governments, capital and technological development involved in the opening up of new resource frontiers in outer space. While debates about off-world mining are often concerned with whether it will ‘succeed’ or not, we are more interested in what it tells us about contemporary frontier-making and what the rendering of extreme environments ever more legible to state and capitalist interests means for resource politics and struggles in the decades ahead. The passing of the SPACE Act – otherwise known as the Commercial Space Launch Competitiveness Act – by the United States government in 2015, posed a fundamental challenge to the principles of the 1967 UN Outer Space Treaty. By allowing US citizens and companies to legally “engage in the commercial exploration and exploitation of space resources” (US Congress, 2015), the SPACE Act marked a potential watershed moment in the legal status of off-world resources, which were previously protected from private or national exploitation as a “common heritage of all [hu]mankind” (United Nations, 1967). Following this controversial move, many other countries have followed suit, with equivalent laws created in Luxembourg, Japan, China, Russia and India. The opening up of space regulation reflects and supports a growing and increasingly ambitious array of space exploration and colonisation programmes, which has seen the entry of new protagonists alongside national governments such as Elon Musk and Jeff Bezos from the billionaire class, as well as asteroid mining companies such as Planetary Resources.
What is behind this accelerating interest in extending human activity into outer space? One explanation lies in recent advances in space technology and especially the potential for robotics and autonomous systems (RAS) to vastly extend the ability of humans to function in outer space (Gao & Chien, 2017). This includes the possibility of opening new resource frontiers, which presents a tantalising prospect for state and capitalist interests. Human history has always been shaped by the actions of powerful societies to expand control over new resource frontiers, as a means of fuelling economic activity and accumulation, and consolidating political power (e.g. Klinger, 2018; Li, 2014; Moore, 2015). The New Space Race is heavily tied up with narratives of a ‘new gold rush’ in strategically important rare metals and minerals, and the development of the raw materials that could enable more permanent human presence on other planets (Pearson, 2018; Valentine, 2012). Historically, frontier expansions have been achieved in various ways, including colonial conquest, capital investment, new legal regimes and the use of forced labour, but also through technologies that extend the ability to exert power at a distance, extract value and transport raw materials. Scientific and technological revolutions have frequently played a crucial role in frontier-making, by enabling new ways of seeing and knowing resource landscapes, manipulating matter, reorganising and moving through space – in ways that have radically reduced the costs of resource exploitation.
In various contexts on Earth and outer space, automated and robotic technologies provide scope for humans to operate in previously hostile and inaccessible resource geographies, which were physically or financially out of reach (Bellingham & Rajan, 2007; Mueller & van Susante, 2012; Wong et al., 2017; Zlotnikov, 2012). In the case of off-world resource frontiers, including the Moon, Mars, near-Earth asteroids and other celestial bodies, the greatest challenges arguably lie in the limits imposed on the human body and machines by hostile environmental conditions (such as extreme temperatures, pressures, vacuums, gravitational forces, radiation, corrosive atmospheres and very fine dust) and the vast distances involved (which heavily constrain communication and teleoperation). In response, space robotics has long been envisaged as facilitating different kinds of space missions, by carrying out “tasks that people cannot do or that are too dangerous, too laborious, or too expensive” (Freitas & Gilbreath, 1982). The New Space Race and recent geopolitical manoeuvring over the rights to own and sell resources obtained from outer space partly reflect the growing capabilities of space robotics in these areas, which could see off-world resource exploitation become a practical and economic possibility in the coming decades.
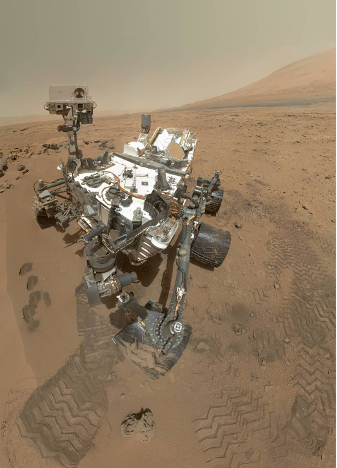
Geared towards overcoming these material barriers, current research and development in space RAS is focused on robotic mobility, manipulation, and autonomous capabilities, as well as operating in extreme environments (Gao & Chien, 2017; Yoshida et al., 2016). Advances in material science and robotic engineering, sensing, perception and navigation systems, machine learning and system-wide autonomy could all be crucial to rendering the outer space frontier legible to new forms of control, territorialisation, intervention and exploitation as sites of resource extraction. The blending together of these different elements is augmenting human capacities in space in three interlocking ways, which could dramatically reduce the costs of colonisation and resource development. First, robots which can materially function in extreme conditions. They can learn about and adapt to their environment and perform autonomous forms of self-monitoring and repair that enable longer-term presence and extend human capabilities to operate in off-world resource landscapes, whether in situ or through robotic proxies at a distance, with minimal risk and cost. Second, through increasing capacities to navigate, move around, sense, measure, survey and process fine-grain environmental and geological data while in situ, space robots offer new ways of seeing and mapping extra-terrestrial frontiers. The production of this kind of knowledge has historically been central to territorial struggles over disputed frontiers, while also rendering resource landscapes knowable and calculable to different forms of intervention and investment (e.g. Klinger, 2018). Third, advances in robotic manipulation, including drilling, handling and processing of raw materials and potential fuel sources, together with automated construction capabilities, could ultimately facilitate the building of physical infrastructure to enable in situ resource development at scale.
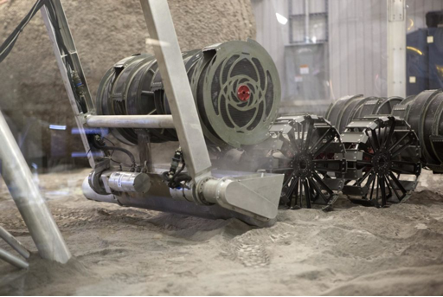
As with previous moments of technological change, RAS is already transforming space by reconfiguring representational and material relations with the frontier. Sitting beneath the struggles of the New Space Race is a growing landscape of ambitious extra-planetary programmes and exploratory missions led by a range of state and private actors, which demonstrate the growing capabilities of space robotics. AI systems are increasingly used on NASA’s Martian rovers to navigate, select rocks and soil for analysis, as well as filtering and prioritising data sent back to Earth (e.g. Bubenik, 2020; Francis et al., 2017). As part of the current Perseverance mission, NASA will soon be carrying out the first ever controlled flight tests on another planet with its Ingenuity Mars Helicopter, which will require a considerable degree of autonomy given the communication delays from Earth. Since January 2019, the China National Space Administration’s (CNSA) Yutu-2 rover has been carrying out a survey on the far side of the Moon (Sekulich, 2020). The solar-powered robot has been using tools such as lunar penetrating radar and imaging spectroscopy to gather information on the Moon’s surface and subsurface geology. These surveys and the recent collection of 2kg of lunar material for analysis back on Earth are expected to yield important data for lunar mining prospects, and the Lunar Exploration Program’s broader objective of establishing a Moon base. In December 2020, the Japan Aerospace Exploration Agency’s Hayabusa2 returned samples from the near-Earth asteroid Ryugu, after a six-year, five-billion-kilometre journey (Crane, 2019). Using LIDAR, optical, near-infrared and thermal-infrared cameras, Hayabusa2 was able to map the asteroid’s surface and topography, before launching four small robotic landers, which could ‘hop’ short distances across the low-gravity terrain to collect different surface-based measurements and imagery. Finally, the probe descended and collected samples remotely blasted out of Ryugu’s surface and subsurface. While not explicitly about mining, Hayabusa2 – like NASA’s equivalent OSIRIS-Rex mission which gathered material from Bennu in October 2020 and is expected to return in late 2023 – is demonstrating robotic systems asteroid mining would necessitate, while providing detailed and valuable geological data.
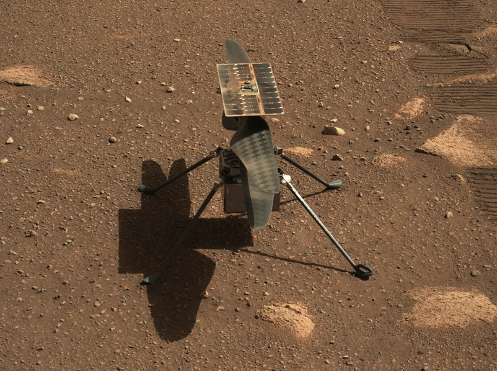
These national programmes also connect to a growing ecosystem of commercial space robotics, financed through government contracts and major private investment capital. Tokyo-based start-up ispace, which has received $125m of private investment to date, aims to achieve the first Japanese Moon landing led by a private entity in 2022 (Etherington, 2020). The company’s longer-term ambition is to “deploy swarms of rovers to the lunar surface to pioneer the discovery and development of lunar resources, enabling the steady development of lunar industry and human presence on the Moon”. Commercial space robotics continues to be developed in the US through new entrants such as OffWorld and long-standing NASA contractors Honeybee Robotics. Technology transfer between on-world and outer space applications is an important aspect of these commercial ventures. OffWorld’s business model is about developing ‘universal’ mining and construction robots which can first be sold and tested for terrestrial use. Honeybee Robotics has worked for the oil, gas and mining sectors for decades, providing “systems for autonomous drilling, automated sampling, in-situ analysis, geotechnical characterization and remote access”. First Mode is developing and adapting space-based robotic systems for use in terrestrial mining – including a $13.5m contract with Anglo American working on its FutureSmart Mining Initiative (Boyle, 2019, 2020). The crossover of RAS technologies and expertise being developed for mining in space and on Earth is particularly stark, but is still largely unexplored in critical research.
The space industry continues to grow, as technological development progresses and the costs of entry for exploration and other missions decline. Nevertheless, despite a proliferating landscape of space robotics innovation and off-world experimentation, a viable programme of commercial exploitation of outer space resource frontiers remains at best decades away – if indeed it ever proves possible. The asteroid mining bubble may already have burst. Both Planetary Resources and Deep Space Industries were sold and wound up in 2019, having failed to generate enough revenue or long-term investment (Abrahamian, 2019). There is currently no practical solution to the logistical problem of creating a cost-effective infrastructure for commercial-scale raw material extraction, processing and transportation to potential buyers. Similar difficulties arise with the costs of launching and transporting significant amounts of material and machinery from Earth for use in outer space. This is driving the focus on using robots for ‘in situ resource utilization’ as a way of sourcing the materials, fuels and feedstocks for longer-term off-world infrastructure and settlements from space itself. Although policy frameworks have been advanced in recent years that may facilitate future enclosures, technical challenges still translate to enormous financial risks, where operational failures with very expensive machinery will almost certainly lead to unrecoverable losses.
Off-world robotic mining may or may not ultimately succeed. Yet there are a range of economic and extra-economic logics driving investment, research and development in space robotics which will have far-reaching implications. Space robotics is part of a broader reworking of resource frontiers on Earth and outer space as transferable automation technologies are applied and developed for different contexts, intensifying and extending possibilities for capitalist extractivism. Technological development of a wider ecosystem of RAS – which augment of human capacities to see, know and intervene in geophysical and biophysical landscapes – will continue to stretch frontier-making possibilities. This has effects on the calculus of power, especially around a set of resources increasingly categorised as essential and strategic raw materials for advanced digital, low-carbon and military technologies. This is clearly reflected in the new geopolitics of space regulation, with the renewed contestation of off-world territory hinging partly on the growing affordability of space travel and related revaluation of extra-terrestrial resources. A key area of critical research is therefore the nexus of relationships between technology, regulation, capital and the exercise of geopolitical power across terrestrial and extra-terrestrial territories that were previously beyond the reach of significant human exploitation.
References
Abrahamian, A. A. (2019, June 26). How the asteroid-mining bubble burst. MIT Technology Review. Accessed here.
Bellingham, J. G., & Rajan, K. (2007). Robotics in Remote and Hostile Environments. Science, 318(5853), 1098–1102. Accessed here.
Boyle, A. (2019, December 18). First Mode forges $13.5M agreement with Anglo American to develop mining tech. GeekWire. Accessed here.
Boyle, A. (2020, September 10). First Mode wins $1.8M contract to build hardware for Psyche mission to asteroid. GeekWire. Accessed here.
Bubenik, T. (2020, June 25). Mars Rover Mission Will Use AI to Help Scientists Sort Out Findings. Courthouse News. Accessed here.
Crane, L. (2019, February 20). Japan’s Hayabusa 2 may finally kick-start the asteroid mining era. New Scientist. Accessed here.
Etherington, D. (2020, August 20). Moon lander startup ispace raises $28 million and launches a new lunar data platform. TechCrunch. Accessed here.
Francis, R., Estlin, T., Doran, G., Johnstone, S., Gaines, D., Verma, V., Burl, M., Frydenvang, J., Montaño, S., Wiens, R. C., Schaffer, S., Gasnault, O., DeFlores, L., Blaney, D., & Bornstein, B. (2017). AEGIS autonomous targeting for ChemCam on Mars Science Laboratory: Deployment and results of initial science team use. Science Robotics, 2(7). Accessed here.
Freitas, R. A., & Gilbreath, W. P. (1982). Advanced Automation for Space Missions. NASA. Accessed here.
Gao, Y., & Chien, S. (2017). Review on space robotics: Toward top-level science through space exploration. Science Robotics, 2(7). Accessed here.
Klinger, J. M. (2018). Rare Earth Frontiers: From Terrestrial Subsoils to Lunar Landscapes. Cornell University Press.
Li, T. M. (2014). Land’s End: Capitalist Relations on an Indigenous Frontier. Duke University Press.
Moore, J. W. (2015). Capitalism in the Web of Life: Ecology and the Accumulation of Capital. Verso.
Mueller, R. P., & van Susante, P. J. (2012). A Review of Extra-Terrestrial Mining Robot Concepts. Earth and Space 2012. Accessed here.
Pearson, E. (2018, December 11). Space mining: The new goldrush. BBC Science Focus Magazine. Accessed here.
Sekulich, D. (2020, April 28). The next frontier: Prospecting and mining on the moon. MINING.COM. Accessed here.
United Nations. (1967). Treaty on Principles Governing the Activities of States in the Exploration and Use of Outer Space, including the Moon and Other Celestial Bodies. United Nations Office for Outer Space Affairs. Accessed here.
US Congress. (2015). U.S. Commercial Space Launch Competitiveness Act. Accessed here.
Valentine, D. (2012). Exit Strategy: Profit, Cosmology, and the Future of Humans in Space. Anthropological Quarterly, 85(4), 1045–1067. Accessed here.
Wong, C., Yang, E., Yan, X., & Gu, D. (2017). An overview of robotics and autonomous systems for harsh environments. 2017 23rd International Conference on Automation and Computing (ICAC), 1–6. Accessed here.
Yoshida, K., Wilcox, B., Hirzinger, G., & Lampariello, R. (2016). Space Robotics. In B. Siciliano & O. Khatib (Eds.), Springer Handbook of Robotics (pp. 1423–1462). Springer International Publishing. Accessed here.
Zlotnikov, D. (2012). Mining in the Extreme. CIM Magazine, 7(5), 50–56.
Andy Lockhart is a research associate at the University of Manchester Sustainable Consumption Institute, working on digital platforms and the future of urban mobility. He is also a visiting researcher at the University of Sheffield Urban Institute where his research focuses on the 'new ecologies of automation'.
Aidan While is co-director of the University of Sheffield Urban Institute, leading its research programme on urban automation and robotics.
Simon Marvin is Professor at the University of Sheffield Urban Institute, where he leads research on the theme of controlled environments.